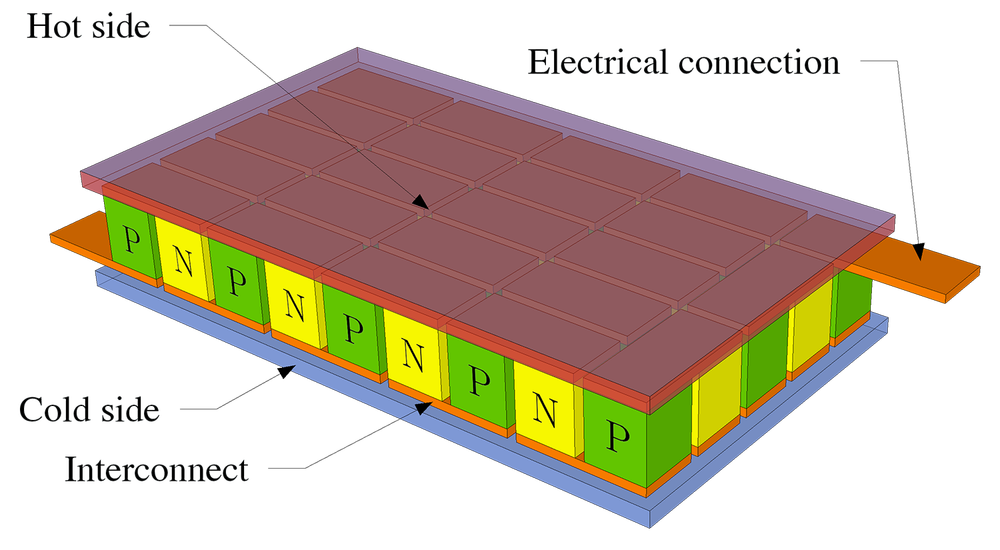
Schematic of a Peltier device. On top and bottom insulating heat spreaders are used and assumed to be connected to heat and cold reservoirs, respectively. They are shown semi-transparent. P- and n-type thermoelectric materials are stacked electrically in series and thermally in parallel. Depending on whether a current source or a resistive device is connected to the electrical connections, this can work as a Peltier element or a thermoelectric generator. (Source: https://commons.wikimedia.org/wiki/File:Peltierelement.png)
ALTOONA, Pa. — Depletion of the earth’s ozone layer, what the Environmental Protection Agency calls our “sunscreen,” has been a major source of environmental concern for decades. In 1987, countries came together to sign the Montreal Protocol, “an international treaty designed to protect the ozone layer by phasing out the production of numerous substances that are responsible for ozone depletion,” including chlorofluorocarbons (CFCs), which were used for cooling and heating. In October 2016, nearly 30 years later, an amendment to the Montreal Protocol specifically addressed the use of hydrofluorocarbons (HFCs), which are “super-polluting, powerful greenhouse gases” and were adopted in part to replace chlorofluorocarbons.
These are positive steps in reducing the number of chemicals that impact the Earth’s ozone layer. But humans still want refrigeration, air conditioning and heating, so the task at hand is to figure out ways to heat and cool without using dangerous substances.
According to Kofi Adu, professor of physics at Penn State Altoona, people all over the world are working on this problem. And it’s complex. What is needed is something capable of heating or cooling, and for that scientists are working with what are known as “thermoelectric materials.”
Adu explained that when you have “two dissimilar materials,” one with “holes” as dominant carriers and the other with electrons as dominant carriers, “you put them together and make one end cold and the other end hot, then electricity starts to flow”—this is known as the Seebeck effect. And, "if you take the same material and pass current through it, one end gets hot and one end gets cold”—this is known as the Peltier effect. It is in these thermoelectric materials that researchers believe they will find the answer to heating and cooling without threatening the ozone layer.
It only makes sense that researchers are looking for the most efficient thermoelectric materials — that’s where it gets complicated. “If we can generate electricity that way or we can make a refrigerator that way, why don’t we have it?” Adu asked. “The difficulty is that there are three parameters that determine the ‘figure of merit’: the Seebeck coefficient, electrical conductivity, and thermal conductivity. These three parameters depend on each other. When you try to improve one, the others start to deteriorate. You have to fine-tune the ‘Goldilocks zone’ where this works. We want to find the best parameters by increasing the figure of merit.”
“Currently, the best figure of merit is 1," he said. "But for any application we need something that is greater than 2 or more. The goal is to fine-tune parameters so that the figure of merit is higher. We want to do it that way because the figure of merit is directly proportional to the product of electric conductivity and the square of the Seebeck coefficient. We call that the power factor. It’s inversely proportional to thermal conductivity.
“The thermal conductivity depends on two contributions: one due to the flow of the charge carriers (electrons) and the vibration of the atoms in the material," he added. "When you increase conductivity, it increases the electron contribution to the thermal conductivity. The only parameter we can play with is the vibration of the atoms (which is the phonons). If we reduce the phonon contribution to the thermal conductivity, that increases the figure of merit.”
It's about reducing the phonon contribution and increasing the power factor, Adu said: “Almost around the world that is what everyone is doing. The problem is so complex. Because of that there are very few materials that have a figure of merit between 1 and 2. We are trying to engineer our materials to increase power while at the same time using larger atoms to decrease thermal conductivity. Adding larger atoms decreases thermal conductivity and so the figure of merit increases.”
The combination that Adu and his student researchers are using to try to increase the figure of merit is Pb-Ge-Se — lead-germanium-selenium. “The thermal conductivity of that material is very low," said Adu. "So we take that material and add it to the material that has a high power factor, forming another composite. We’re taking ordinary materials such as boron doped silicon-germanium and investigating the effect on the figure of merit.
Brianne McFarland, an industrial engineering major now at University Park campus, started working on this problem over the summer with Adu. She describes working on "silicon germanium doped with boron."
"We wanted to look at the thermal conductivity to get the overall thermoelectric property of it," said McFarland. "We were able to measure the power factor because we had the machine [for that measurement]. However, we weren’t able to measure the thermal conductivity because we didn’t have the machine for it.”
To take a look at those materials, McFarland says, “we made a silicon germanium pellet. Then we crushed that and added the boron and made another pellet.” The crushing and mixing were completed with either a mortar and pestle or a ball mill; then the materials were pressed together with an arbor press. “After we pressed it, we had to bake it at 1200°C for four hours." That's the temperature at which it would form an alloy.
“Then we brought it to the Materials characterization Lab at the Millennium Science Complex at University Park to measure the resistivity and the power factor. For thermoelectric materials you want a high electrical conductivity and lower thermal conductivity. That’s what we were trying to get. Silicon germanium had high electrical conductivity and lead-germanium-selenide had low thermal conductivity. Professor Adu thought if we would try to combine the two we might get the properties we want.”
When asked how she liked the work, McFarland says, “It was very interesting. It’s not something I ever thought about or was interested in, but it actually turned out pretty cool. We didn’t get the results we wanted (because we couldn’t do the test) but we looked at the power factor, which compared to silicon-germanium decreased but compared to lead increased. Lead really influenced the silicon-germanium.”
"Why is this important? Refrigerators and air conditioners use CFCs," explained Adu. "You can design a refrigerator with no moving parts and that does not emit any harmful chemicals. We can also use this to harvest electricity.”
But the goal is to make the technology work better. While “BMW has done a lot of work to create this in their cars, to warm up a car before you even start it,” Adu said, we won’t see significant results in heating and cooling for another 10–20 years. For now, people will have to settle for small steps, like wine bottle jackets that cool wine quickly.